pp 1‐18
Hari Kumar Singh
Centre of Excellence Renewable & Sustainable Energy Studies, Suresh Gyanvihar University, Jaipur, Rajasthan, India
D. Buddhi
Centre of Excellence Renewable & Sustainable Energy Studies, Suresh Gyanvihar University, Jaipur, Rajasthan, India
Abstract—A move to effectuate a secure and cozy state of environment has always been one of the prime obsessions of the human desire. Additionally, it is of critical significance to study adverse effects (global warming, pollution, e.t.c) of fossil fuels (still being a larger contributor of power supply among all energy sources) on the environment Phase change materials used for latent heat storage systems have a high energy density and show an isothermal behavior during the heat retrieval process, provide a great potential for various thermal energy storage applications for the desired range of temperature. This paper reviews previous work carried out by different researchers focusing on properties of phase change materials for sub-ambient temperature applications. This paper also looks at the problems associated with the heat transfer enhancement, compatibility, sub-cooling and degradation of phase change materials after using finite number of charging-discharging cycles.
Keywords—Phase change material, Heat transfer enhancement, Thermal energy storage, Latent heat.
- INTRODUCTION
In recent decades energy efficiency has been gaining high attention since energy crises took place in 1970. In view of demanding continuous and smooth power supply by the people, with elevating living standards and having a considerable share in continuous increasing population has also turned energy efficiency up becoming a key concern. Moreover, challenges are also being faced in meeting the peak load requirement which result in large demand and supply mismatch. Various factors concerning neutralization of demand and supply mismatch developed by the author are shown in Fig. 1.
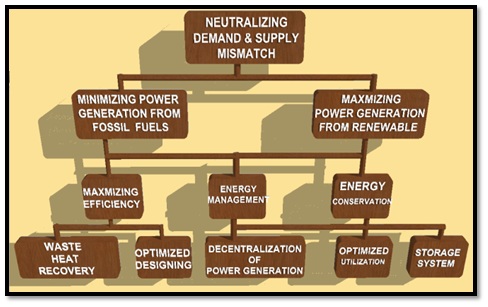
factors concerning neutralization of demand and supply mismatch
Fig. 1. An overview of factors concerning neutralization of demand and supply mismatch [Developed by author]
In India, for supplying required power in peak hours and high operating cost power generators are used in addition with thermal and hydro power plants. With the same trend the authorities also predicted the energy requirement of 1354874 and 1904861 GWhr with peak load of 199540 and 283470 MW for 2016-17 (at 12th five year plan end) and 2021-22 (at 13th five year plan end) respectively [1]. One of the effects of fulfilling the peak load requirement is oversizing of the grid. In 2008 US had generated one trillion watts of electricity which was almost twice of the average electricity used during that year [2]. Also, it brings up an important issue, the people who are still remain untouched approaching electricity, can be benefitted by the surplus power that can be conserved by befitting energy management techniques. To take the benefits of differential tariff, energy security, off peak power utilization and variable renewable energy sources one of the technology used is thermal energy storage. Hence, therefore favorable conditions for thermal storage technology are as follows:
- Thermal storage of building structures [3].
- Domestic water heating and cooling systems [4].
- Space heating and cooling of buildings [5, 6].
- Peak load shifting [7].
2. THERMAL ENERGY STORAGE
TES being reversible nature offers a great opportunity to store desired amount of heat and cold energy that can be retrieved for later use. It can be broadly classified as Thermal and thermochemical heat storage. Furthermore, thermal energy is subcategorized as sensible and latent heat storage.
2.1 Sensible heat storage
When the heat is supplied to a storage medium shows a continuous trend of increasing temperature (heat and temperature can be observed by the sensor) and vice versa is considered as sensible heat. Examples of sensible hest storage mediums are air, water, oil, sand, rock beds, bricks etc. Amount of sensible heat stored in the mass of the storage material y (Process shown in Fig. 2.) is defined as follows:
Qs = m cp (TE – TA)
Where,
cp = Specific heat at constant pressure of the storage material [kJ/kg.K],
m = Mass of the storage material [kg],
T = Temperature storage material [K],
There are large applications of sensible heat storage, Dincer and Rosen [8], Hadorn [9] and Paksoy [10] studied and described various technologies of sensible heat storage in the literatures.
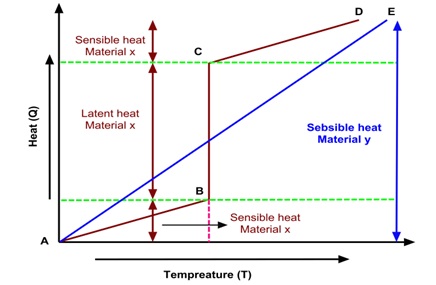
Families of phase change materials
Fig. 2. Temperature and heat variations of sensible and latent heat storage of materials x and y during charging-discharging process.
2.2 Latent heat storage
Latent heat is defined as heat absorbed or rejected by PCMs during transformation of phase (solid-solid, solid-liquid, liquid-vapor and vice versa) at constant or nearly constant temperature . Amount of total heat stored (sensible heat + latent heat) in the mass of the storage material x (Process shown in Fig. 3.) is defined as follows:
QT = (QS)AB + (QL)BC + (QS)CD
Or descriptively,
QT = m cp (TB – TA) + m ∆hCB + m cp (TD – TC)
Where,
∆hCB = Latent heat of fusion per unit mass of the storage material [kJ/kg],
The following figure shows temperature and heat variations for sensible (material x) and latent heat storage (material y) materials during heat transfer process. Charging of thermal storage for material x process A to B denotes sensible heat addition (continuous increase in temperature), B to C latent heat absorption (constant temperature), C to D sensible heat addition (continuous increase in temperature). Charging of thermal storage for material y process A to B denotes only sensible heat absorption.
3. PHASE CHANGE MATERIALS
There are large numbers of phase change materials available with melting point at the desired range of temperature which directly matches with various applications. PCMs can be classified as shown in Fig. 3:
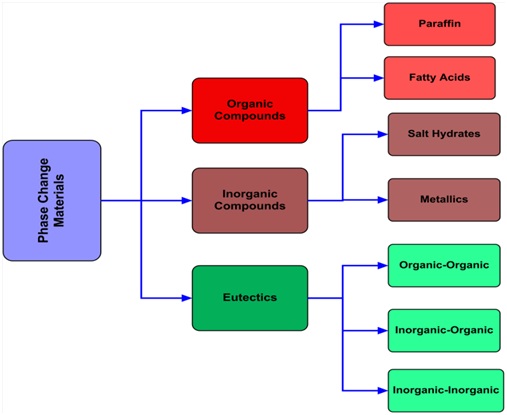
Families of phase change materials
Fig. 3. Families of phase change materials [11].
3.1 Organic phase change materials
Organic PCMs can be classified as paraffin and non paraffins which includes following properties. Organic materials are widely used for various applications because they possess congruent melting without phase segregation (self nucleation i.e. crystallization with little or no supercooling) and generally non corrosive in nature.
3.1.1 Paraffins
Paraffin PCMs mostly contains straight chain normal alkanes. Large amount of heat is released during crystallization of (CH3–) chain. With increase in number of carbon atoms melting point temperature and latent heat of fusion also increases. Being noncorrosive in nature they are very stable up to a temperature of 500 0C. Paraffins also have been a center of attraction from long time for suitability in large number of applications because of their availability in large range of temperature. Additionally they also have low vapor pressure in molten form with cost effective and reliable in nature. Peng et al. [12] discussed different paraffin waxes which varies in latent heat from 128–198 kJ/kg and melting point temperature from 12 to 71 oC.
3.1.2 Non-paraffins
Unlike paraffins non-paraffin PCMs have their own distinct properties. These materials cannot be used for those applications which require direct exposure to very high temperature. They are also subcategorized into fatty acids and non-paraffins. Buddhi and Sawhney [13] described large number of organic PCMs used for various applications of thermal storage such as alcohol , glycol and esters .
3.2 Inorganic phase change materials
Inorganic PCMs are categorized as metallics and salt hydrates. They have high latent heat of fusion and widely available in large range of temperature for variety of thermal storage application. Apart from these advantages they possess high degree of supercooling and actively prone to corrosion and rusting specially with metals [14, 15]. According to the various literature it is also explained that many inorganic PCMs are considerable inexpensive for many Thermal storage applications [16]. The general formula of salt hydrates is AB.nH2O which represents alloy of water forming crystalline solid and inorganic salt.
3.3 Eutectics
A minimum melting composition of two or more components, which during crystallization congruently freeze and melt to form a mixture. They provide a little or almost no opportunity for phase segregation during melting and freezing process. Sharma et al. [15] mentioned in that in eutectics there is no segregation occurred during phase cane. Commonly, Inorganic–Inorganic, Organic–Organic and Organic–Inorganic eutectics PCMs are used for various applications.
4. THERMO-PHYSICAL PROPERTIES OF CANDIDATES PCMs
Thermo-physical properties of low and medium temperature investigated Candidates PCMs are described as organic, organic mixture, paraffins, non paraffins, fatty acids, fatty acids and mixture, inorganic (hydrated salt), inorganic mixture, inorganic, inorganic compounds, salt hydrates, eutectics, eutectic organic ,eutectic inorganic, metallics and water in ascending order of temperature respectively, are shown in Table 1.
Table 1. Thermo-physical properties of low and medium temperature investigated Candidates PCMs
Materials | Types | Melting temperature (oC) | Heat of fusion (kJ/kg) | References |
Dodecane | Organic | -9.6 | 216 | [17] |
Triethylene glycol Organic | Organic | -7 | 247 | [18] |
Microencapsulated
94% tetradecane + 6% tetradecanol |
Organic | 5.1 | 202.1 | [19] |
Microencapsulated
100% tetradecane |
Organic | 5.2 | 215 | [19] |
Microencapsulated
96% tetradecane + 4% Tetradecanol |
Organic | 5.2 | 206.4 | [19] |
Bulk 100% tetradecane | Organic | 5.5 | 215 | [19] |
Bulk 96% tetradecane
+ 4% tetradecanol |
Organic | 5.5 | 206.4 | [19] |
Bulk 94% tetradecane
+ 6% tetradecanol |
Organic | 5.5 | 202.1 | [19] |
Paraffin C14 | Organic | 5.5 | 228 | [20] |
Tetradecane (91.67 mole.%)
+hexadecane (8.33 mole.%) |
Organic | 5.9 | 258 | [21] |
n-Tetradecane | Organic | 6 | 230 | [22] |
Lauryl alcohol–Caprylic acid
(2:3 by quality) |
Organic | 6.2 | 173.2 | [23] |
Caprylic acid–Palmitic acid
(9:1 by quality) |
Organic | 6.54 | 116.5 | [24] |
Dodecanol–Caprylic acid
(40.6:59.4 by quality) |
Organic | 7 | 178.6 | [24] |
Paraffin C15–C16 | Organic | 8 | 153 | [22] |
Polygycol E400 | Organic | 8 99. | 6 | [22] |
4.1 PCM selection criteria
In the designing of thermal storage system, PCMs must be selected which contain desirable thermodynamic, kinetic, chemical, technical, and economic characteristics otherwise it may lead to low efficiency and high economic losses. Some of the criteria considered in evaluating PCMs are mentioned as follow: [26]
4.1.1 Thermal, chemical, technical & economic criteria
- PCM should have a melting point at the required operating temperature.
- It must possess a high latent heat per unit volume so that desired quantity of energy can be availed from minimum PCM volume.
- PCM should possess long term chemical stability.
- It should be noncorrosive with the enclosure.
- It should be simple in use.
- It should possess suitable applicability.
- Its effectiveness should be high.
- It should available in the large scale which is ready to use with little or no required modifications.
5. FACTORS AFFECTING THERMOPHYSICAL PROPERTIES OF PCMs
5.1 Thermal stability of PCMs at low temperatures
For a long life and reliable output of thermal storage system one of the basic requirements is that there should be insignificant or no degradation in the thermophysical properties of PCMs after undergoing a large number of charging-discharging cycles. Tyagi and Buddhi [27] investigated thermo physical properties of calcium chloride hexahydrate ( phase change temperature of 29 oC) with SrCl2.6H2O (0.5 %) as a nucleating agent in charging-discharging test for 1000 cycles .
5.2 Phase segregation and subcooling of PCM at low temperatures
When a PCM, especially salt hydrates, during solidification process, crystallizes at a temperature which is significantly below its freezing point temperature is termed as subcooling or supercooling. If the fraction of heat released during heat extraction (solidification) process is more than the sensible heat lost then its temperature rises to melting point temperature and remains their until completion of phase change of PCM ( Shown in Fig. 4a) . If opposite of the above explained process happens then there is a possibility that the temperature will not rise to its meting point temperature (Shown in Fig. 4b).
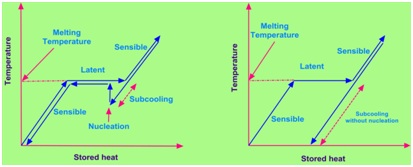
Effect of subcooling on heat storage
(a) (b)
Fig. 4 Effect of subcooling on heat storage.(a) subcooling with nucleation and (b) subcooling without nucleation. [26]
6. HEAT EXCHANGER CONFIGURATIONS FOR PCMs
For desired rate of charging and discharging of PCMs in latent heat thermal storage systems demand good heat transfer between PCM and cooling medium. Teggar et al. [28] conducted numerical study on solidification of PCMs (water/ice) of the parallel plate type heat exchanger using a conduction model.
6.1 Extended fins enhancement process
In view of ease of fabrication ,simplicity and low cost considerations, fins as an extended surfaces, are widely used to increase the heat transfer surface area which results in better efficiency. Lamberg et al. [29] analytically analyzed solid–liquid interface location and temperature distribution of the fin in a solidification process with constant end-wall temperatures in a finite PCMs (Thermophysical properties shown in Table 2.
Table 2. Physical properties of the phase change and the fin materials. [29]
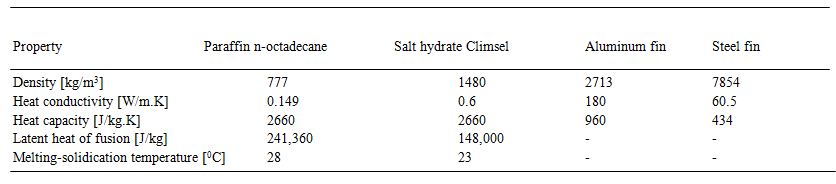
conducted experiment on solar vapor absorption
Agyenim et al. [30] conducted experiment on solar vapor absorption (Li-Br/water) air conditioning system, in which circular tubes with longitudinal fins (Shown in Fig. 5) was applied as a heat exchanger (erthertoal PCMs).
Fig. 5. Cross section view of Shell and tube type heat exchanger (A) without fins
(B) circular fins and (C) longitudinal fins [30].
6.2 Encapsulation of PCMs for sub-ambient temperature applications
In the indirect contact type heat transfer processes, between PCM and heat transfer fluid, it is required to keep PCM in the separate enclosure. The above mentioned method is known as encapsulation has been widely using in laboratory research work as well as commercial thermal storage systems because of the various advantages.
6.2.1 Microencapsulation of PCMs
In micro-encapsulation the PCM particles (particles size ranges from 1 to1000 µm) are enclosed inside a very thin material of desired physical and chemical properties. In the micro-encapsulation technique the shape and size of the composite system (PCM and encapsulation) may vary according to the usage for desired applications
6.2.2 Macroencapsulation of PCMs
In macro-encapsulation The PCMs are enclosed inside the containments of different sizes (containments may vary in capacity ranging from milliliters to several liters of PCM) and shapes (spheres ,tubes, bags, panels ,matrices, pouches or other shapes containers) as desired for different applications. According to Li Xiaoyan et al. [31] , at the end of solidification process of PCM freezing rate slightly increases, as energy required for phase transformation liquid fraction of PCM reduces. In the freezing process of PCM inside spherical shell , fraction of solid PCM starts floating at the top due to the difference in density of solid-liquid masses (also known as buoyancy effect), termed as unfixed melting, shows actual phase transition behavior in practical situations the Fig. 6 shows difference in unfixed and fixed melting.
Fig. 6. Schematic view of a spherical capsule (a) freezing (b) fixed melting (c) unfixed melting [31].
7. SPACE COOLING WITH PCM
Thermal storage is used in air conditioning system to stabilize the operation and utilize its advantage during off peak hours. Studies are basically concentrated on Ice and eutectic salts as a PCM but, as low temperature heat exchange is not desirable for air conditioning purposes, eutectic salts (available in wide range of heat transfer temperature) are certainly preferred to ice storage systems. Wang et al. [32] experimentally analyzed heat transfer rate and storage capacity of a central air-conditioning system with ice ball as a PCM and the glycol solution as heat transfer fluid shown in Fig. 7. They found that the major storage (around 81% of total storage capacity) of thermal energy was exploited from PCM.
Fig. 7. Picture of balls before and after crystallization used in the air conditioning system [32].
Some commercial thermal energy systems with technical details for space cooling are described in Table 3.
7.1 Case studies of sub-ambient temperature TES systems in buildings
Apart from laboratory experiments and mathematical modeling the performance of a thermal storage system can only be analyzed correctly in actual sites where the system is installed. Some of the studies based on performance analysis at practical sites are mentioned in the Table. 4.
8. CONCLUSION
This review focuses on different properties of PCM for sub-ambient temperature applications. The role of thermal energy storage systems for eliminating gap between power demand and supply has been discussed. For designing of efficient latent heat storage systems selection of correct PCM is very important. Hence, therefore various properties associated with criteria of PCM selection such as thermodynamic, kinetic, chemical, technical and economic criteria are well discussed in this review paper. The influence of extended fins, metal insertion and encapsulation of PCM on the performance of latent heat thermal storage is also studied. Moreover, having reviewed all the literatures presented in this paper, based on PCM for latent heat storage systems, it is found that a very less work has been done on low temperature thermal storage applications that need to be focused more rigorously in future to exploit its complete potential. Additionally, it has been also observed that the high cost of PCMs is still a suppressing factor in commercializing PCM based storage systems in the market that makes these applications away from the reach of the common people.
Table 3. Thermal energy systems for space cooling.
Table 4. Different case studies of low temperature thermal storage systems [33].
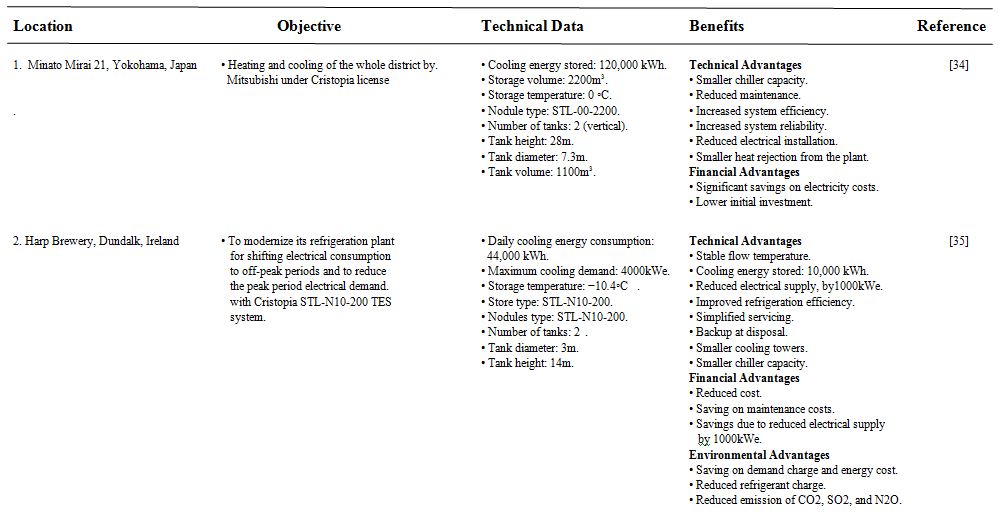
Different case studies of low temperature thermal storage systems
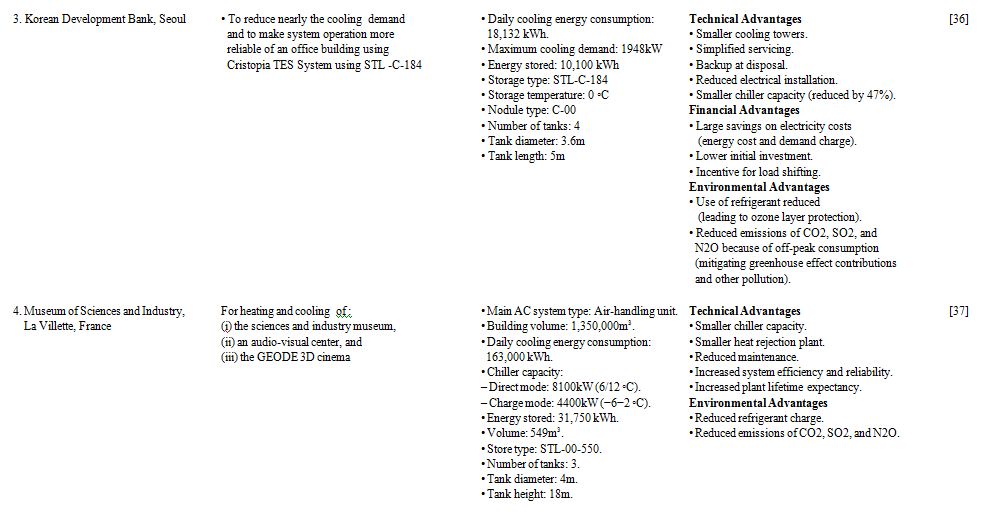
Three case studies of low temperature thermal storage systems
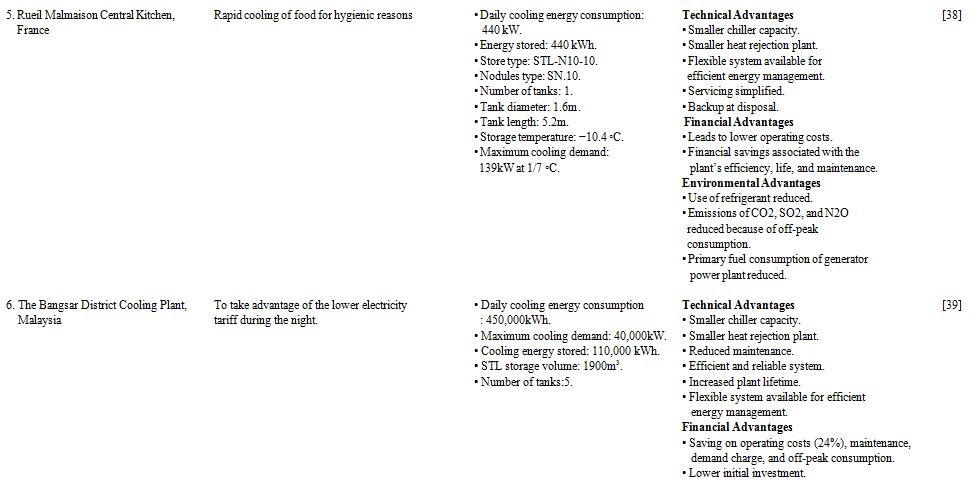
Two case studies of low temperature thermal storage systems

Different case studies of low temperature thermal storage systems – 7
References
- National Electricity Plan U/S 3 (4) of Electricity Act 2003, Volume 1, Government of India Ministry of Power Central Electricity Authority, January 2012.
- “Electric Power Annual 2000” (with data from 2008). U.S. Energy Information Administration.
- Pasupathy A, Athanasius L, Velraj R, Seeniraj RV. Experimental investigation and numerical simulation analysis on the thermal performance of a building roof incorporating phase change material (PCM) for thermal management. Applied Thermal Engineering 2008;28:556–65.
- Bony J, Citherlet S. Numerical model and experimental validation of heat storage with phase change Energy and Buildings 2007;39:1065–72.
- Castell A, Martorell I, Medrano M, Pérez G, Cabeza LF. Experimental study of using PCM in brick constructive solutions for passive cooling. Energy Build 2010;42:534–540.
- V. Tyagi, D. Buddhi, PCM thermal storage in buildings: a state of art, Renewable and Sustainable Energy Reviews 2007;11:1146–1166.
- Hasnain SM. Review on sustainable thermal energy storage technologies, part II: Cool thermal storage. Energy Conversion and Management 1998;39(11):1139–1153
- Dincer İ, Rosen MA. Thermal energy storage: systems and applications. Chichester, England: Wiley; 2002.
- Hadorn J.-C. (ed.) Thermal energy storage for solar and low energy buildings. Servei de Publicacions (UDL), Lleida (2005) ISBN 84-8409-877-X.
- Paksoy H.Ö. (ed.): Thermal energy storage for sustainable energy consumption – fundamentals, case studies and design. Springer, (2007), NATO Science series II Mathematics, Physics and Chemistry – 234, ISBN 978–1–4020–5289–7.
- Zhou D, Zhao C.Y, Tian Y. Review on thermal energy storage with phase change materials (PCMs) in building applications. Applied Energy 2012 ; 92: 593–605.
- Peng S, Fuchs A, Wirts RA. Polymeric phase change composites for thermal energy storage. J Appl Polym Sci 2004;98:1240–51.
- Buddhi D, Sawhney RL.In: Proceedings on thermal energy storage and energy conversion; 1994
- Farid M, Khudhair M, Razack K, Said Al-Hallaj. A review on phase change energy storage: materials and Energy Conversion and Management 2004;45:1597–615.
- Sharma A, Tyagi V, Chen C, Buddhi D. Review on thermal energy storage with phase change materials and applications. Renewable and Sustainable Energy Reviews 2009;13:318–45.
- Lane G.A. et al. Macro-encapsulation of PCM. Report no. ORO/5117-8. Midland, Michigan: Dow Chemical Company; 1978. p. 152
- Kenisarin MM. High-temperature phase change materials for thermal energy storage. Renew. Sustain. Energy 2010;14:955–70.
- Cabeza LF, Castell A, Barreneche C, de Gracia A, Fernández AI. Materials used as PCM in thermal energy storage in buildings: a review. Renewable and Sustainable Energy Reviews 2011;15:1675–95.
- Alvarado JL, Marsh C, Sohn C, Phetteplace G, Newell T. Thermal performance of microencapsulated phase change material slurry in turbulent flow under constant heat flux. International Journal Heat Mass Transfer 2007;50:1938–52.
- Saito A. Recent advances in research on cold thermal energy storage. International Journal of Refrigeration 2002;25:177–89.
- Dotsenko SP, Martsinkovskii AB, Danilin VN. Thermal storage properties of n-paraffins, fatty acids and multi component systems on their basis In :e-Journal: Fiziko-khimicheskii analiz svoistv mnogokomponentnykh system, 2004, vyp.1(in Russian).Available from /http://www.kubstu.ru/fh/fams/dopoln3.htmS.
- Regin AF, Solanki SC, Saini JS. Heat transfer characteristics of thermal energy storage systems using PCM capsules: a review. Renewal Sustainable Energy Reviews 2008;12:2438–58.
- Wang Shoutao. Investigation on organic phase change materials in energy storage air conditioning system. (Doctor Thesis) Dalian Maritime University, Dalian, Shenyang, China; 2008.
- Xing Lin, Fang Guiyin, Yang Fan. Preparation and thermal performance analysis of cold storage material for air conditioning system. China Appliance 2006;3:47–8.
- Hu Xiaocai, Wu Huijun, Zhou Xiaoqing. Crystallizing characteristics of binary mixtures of dodecanol / caprylic acid for phase change cold storage. Journal of Guangzhou University 2011;10(2):60–3.
- Mehling H, Cabeza, LF. Heat and cold storage with PCM. An up to date introduction into basics and Springers-Verlag Berlin Heidelberg; 2008.
- Tyagi VV, Buddhi D. Thermal cycling testing of calcium chloride hexahydrate as a possible PCM for latent heat storage. Solar Energy Mater Solar Cell 2008;92:891–9.
- Mohamed Teggar, El Hacène Mezaache2, Numerical Investigation of a PCM Heat Exchanger for Latent Cool Storage, Energy Procedia 2013; 36: 1310 – 1319.
- Piia Lamberg, Kai Siren. Approximate analytical model for solidification in a finite PCM storage with internal fins, Applied Mathematical Modelling 2003; 27: 491–513.
- Agyenim F, Eames P, Smyth M. A comparison of heat transfer enhancement in a medium temperature thermal energy storage heat exchanger using fins. Solar Energy 2009;83:1509–20.
- Li Xiaoyan, Ma Zuiliang. Study on thermal performance of PCM for air- conditionaing and infection of unfixed melting problem in sphere. Acta Energiae Solaris Sinica 2007;8(8):892–5.
- Wang J, Wang S, Zhou Y. Study on ice storage characteristics of small-scale storage tank filled with ice In: Proceedings of the 2010 Asia–Pacific power and energy engineering conference, APPEEC; 2010.
- İbrahim Dinҫer and Marc A. Rosen: Thermal Energy Storage: Systems and Applications, Second Edition; © 2011 John Wiley & Sons, Ltd. ISBN: 978-0-470-74706-3.
- Cristopia (2001a). MM21 in Yokohama (Japan), Cristopia Energy Systems, France, http://www.cristopia.com/english/project/yokohama.html.
- Cristopia (2001b). Harp Guinness Subsidiary (Ireland), Cristopia Energy Systems, France, http://www.cristopia.com/english/project/harpguinness.html.
- Cristopia (2001c). Korean Development Bank (Korea), Cristopia Energy Systems, France, http://www.cristopia.com/english/project/koreanbank.html.
- Cristopia (2001d). Museum of Sciences and Industry: ‘La Villette’ (France), Cristopia Energy Systems, France, http://www.cristopia.com/english/project/lavillette.html.
- Cristopia (2001e). Rueil Malmaison Central Kitchen (France), Cristopia Energy Systems, France, http://www.cristopia.com/english/project/rueil.html.
- Cristopia (2001f). Bangsar District Cooling Plant (Malaysia), Cristopia Energy Systems, France, http://www.cristopia.com/english/project/bangsar.html.
- EPS (2000). Thermal Energy Storage Systems, Environmental Process Systems Limited, Berkshire, http://www.epsltd.co.uk.
- http://www.pcmproducts.net/files/china_shipping_flatice_system_1_.pdf
- http://www.pcmproducts.net/files/ZEO_Malaysia_Project.pdf
- http://www.pcmproducts.net/files/passive_cooling_catalogue.pdf
- http://www.veslpcm.com/block-vesl/
- http://www.veslpcm.com/microvesl-engineered-sphere/
- http://www.veslpcm.com/macrovesl-multi-chambered-sphere/